Lab 4 Protocols
- Examine slabs through human brainstem specimens
- Learning objective: to recognize the principal features of the brainstem that are visible with the unaided eye, including the general location of cranial nerve nuclei and the neuromodulatory nuclei.
- Specimens: whole brain slabs or hindbrain slabs cut through the brainstem
- Activities:
- Refer to Figures 3.5–3.7 and identify the regions in wet slabs through the human brainstem containing cranial nerve nuclei (refer also to the Brainstem Atlas in Sylvius4 Online).
- Refer to Figures 3.11 & 3.14 and see if you can find the “black substance” in the midbrain and pons.
- Predict the impairments with brainstem stroke
- Learning objective: to discuss functional impairments associated with vascular lesions that are typical
in the brainstem.
- Resources: brain or brainstem models; Sylvius4 Online
- Activities:
- Work through Challenge 3.1 by referring to Figure 3.8 and the charts and figures associated with
descriptions of various sections through the medulla, pons and midbrain
- Refer to the Brainstem Atlas in Sylvius4 Online for additional sections and annotations as needed.
- Identify the vessels that supply major medial and lateral regions of the brainstem.
Introduction
Of chief importance in understanding the organization of the brainstem is knowledge of what is localized in each embryological subdivision and in any transverse section. You have already faced the first step toward competency with the essential knowledge—recognition of the external features of each brainstem subdivision, including the associated cranial nerves. Moreover, you were introduced to many important internal gray matter and white matter structures of the brainstem in the last lab chapter. Here, we turn our attention to two categories of gray matter structures that are critical for understanding neurological function and human behavior: the nuclei of the cranial nerves, and the neuromodulatory nuclei of the brainstem. After working through this lab, you should be able to recognize components of important sensory, motor and modulatory systems in each of the embryological divisions of the brainstem.
The cranial nerve nuclei

The cranial nerve nuclei are made up of the neurons in the brainstem that receive primary sensory inputs or that give rise to motor outputs. Just as the spinal cord receives sensory information from the body surface and deeper tissues and sends motor axons to striated muscles and to the autonomic ganglia, similar inputs and outputs exist for the head and neck region. In addition, there are specialized sensory inputs in the head that do not have equivalents in the spinal cord. These include the inputs for hearing, balance and taste. The motor outputs are just a little more complicated as well. There are nuclei that innervate the extrinsic eye muscles and the tongue. Since the muscles that are innervated are derived from somites, these motor neurons are exactly equivalent to those in the ventral horn, which also innervate muscles derived from somites. In addition, there are nuclei in the brainstem that innervate the muscles derived from the branchiomeres of the pharyngeal arches (jaw muscles, muscles of facial expression, the pharynx and larynx). There is only one such cell group in the spinal cord; it innervates the trapezius and sternocleidomastoid muscles, which are also derived from branchiomeres. It is included with the cranial nerve nuclei, since it gives rise to the spinal part of cranial nerve XI. Finally, there are cell groups in the brainstem that form part of the visceral motor (autonomic) system and send axons to autonomic ganglia in organs throughout the body. By working through the Challenges in the last chapter, you have already encountered many of these nuclei; let’s now build a stronger appreciation for the organization of these nuclei in each division of the brainstem recalling some of what you learned about the functions of the cranial nerves in Normal Body.
The organization of the sensory and motor neurons in the spinal cord and brainstem are similar, for reasons that are clear when one considers the development of these two regions of the neural tube—as explained in Figure 4.1. Sensory neurons, which are located dorsally in the spinal cord, are located laterally in the medulla and pons. Motor neurons, which are located ventrally in the spinal cord, are located medially in the medulla, pons, and midbrain.
Nevertheless, the internal organization of the brainstem is considerably more complicated than that of the spinal cord. However, two factors work in your favor as you study its features. First, important general principles of organization of the spinal cord also hold true for the brainstem. Second, much of the complexity of the brainstem is contributed by cell groups and axon tracts that will not be considered in this course.
One further point can be made from Figure 3.1. In the medulla, the motor column that gives rise to somatic motor nuclei also gives rise to the motor neurons that will innervate the branchiomeric muscles. These motor neurons migrate from
the midline into the ventral-lateral part of the tegmentum and send their axons out in nerves that exit the brain laterally
(i.e., V, VII, IX, X, and XI). The somatic motor neurons, on the other hand, send their axons out ventrally (in a line with
ventral roots of the spinal cord), except for the trochlear nucleus (as noted in the previous chapter). Oddly, the
parasympathetic preganglionic neurons (visceral motor neurons) in the medulla and pons also send their axons out
laterally; in the spinal cord (and in the midbrain), the autonomic preganglionic axons leave in the ventral roots. All of the
sensory inputs enter the brain laterally.
If you take the time to appreciate the organization of the cranial nerve nuclei, it will be much easier to learn how the ten
pairs of cranial nerves supply sensory inputs to or derive motor output from the 16 pairs of cranial nerve nuclei. How
do these nuclei relate to the components of the cranial nerves that you studied in Lab 2? Table A2 below lists the cranial
nerve nuclei and sensory ganglia from which the sensory and motor components of each nerve arise.
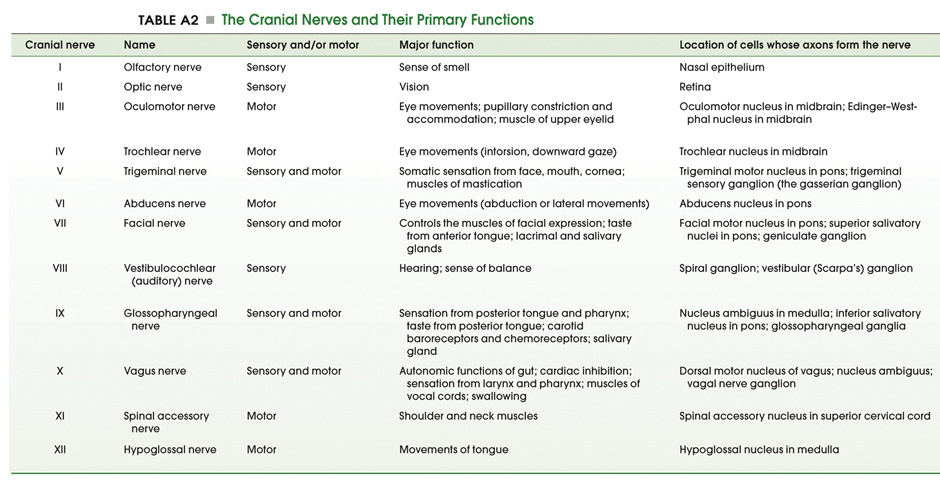
The sixteen cranial nerve nuclei can be most easily remembered if they are assembled into functional groups and anatomical location (Table A3—from Purves et al., Neuroscience, 6th Ed.; Figure 4.2). Three of the groups are motor nuclei: a somatic motor group, a branchial motor group, and a visceral motor (parasympathetic preganglionic) group. The other three groups are sensory nuclei: general sensory, special sensory, and visceral sensory.
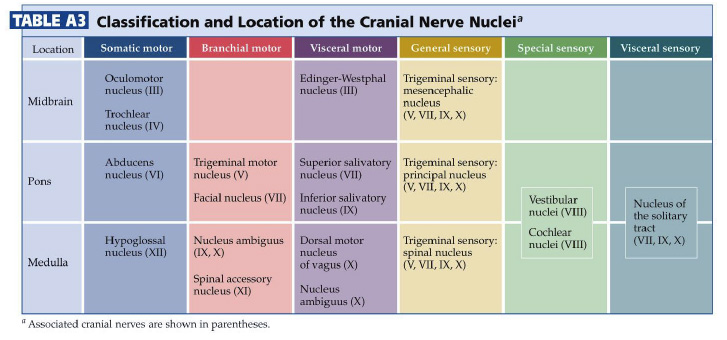
Table A3 illustrates the point that most of the cranial nerves are connected to only one or two cranial nerve nuclei. Only three nerves carry components from more than two nuclei. These nerves—VII, IX, and X—each carry five components: a branchial motor component, a parasympathetic component, a somatic sensory component, a special visceral sensory component (taste), and a general visceral sensory component. These three nerves lack a somatic motor component.
Location of the cranial nerve nuclei
A schematic overview of how these nuclei are arranged in the mature brainstem is presented in Figure 3.2 and 3.3. Three points should be taken from these figure.
- The cranial nerve nuclei lie in the tegmentum of the brainstem, as do many of the major ascending and descending tracts.
- Just as in the spinal cord, the nuclei that receive sensory inputs via the cranial nerves are spatially separate from those that give rise to motor output, with the sensory nuclei located laterally and the motor nuclei located medially
- The cranial nerve nuclei that serve comparable functions are aligned in the longitudinal axis of the brainstem, even when comparable nuclei (e.g., somatic motor nuclei) are distributed across brainstem subdivisions (cf. Figure 4.1). The spatial segregation of sensory and motor functions provides an important clue for localization of focal damage in the brainstem. Thus, an understanding of the functions and locations of cranial nerve nuclei is essential for diagnosing (and treating) neurological injury, dysfunction and disease.
Location of the cranial nerve nuclei in brainstem cross-sections
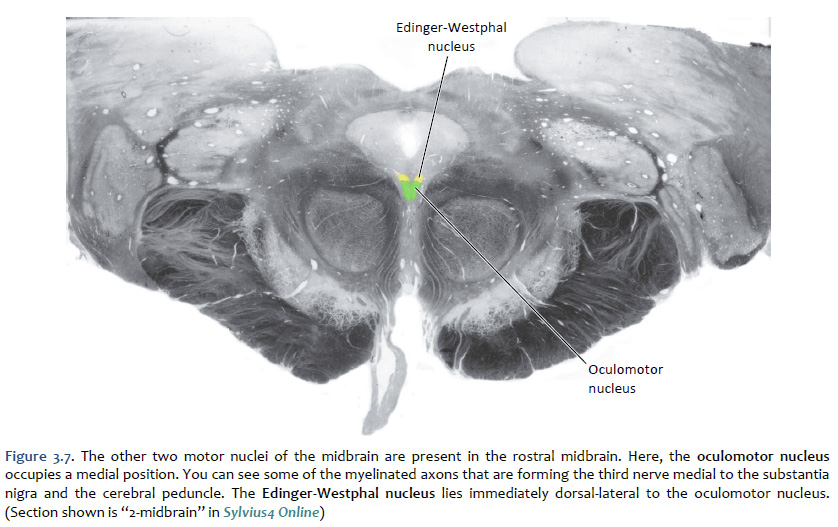
Some of the cross-sections that were introduced earlier are reproduced in Figures 3.4, 3.5, 3.6, and 3.7. The locations of most of the cranial nerve nuclei listed in Tables A2 & A3 are indicated on these sections.
Only four of the cranial nerve nuclei are not identified in the preceding figures. The spinal accessory nucleus is found in the first few cervical segments of the spinal cord. Very few people in the world know just where the superior and inferior salivatory nuclei are located (somewhere in the dorsal tegmentum of the rostral medulla/caudal pons). The cochlear nucleus is located just at the junction of the medulla and pons, where this nucleus wraps around the lateral aspect of the inferior cerebellar peduncle (visible, but unlabeled in Sylvius4 Online, section “8 – Medulla”).
You should now be able to easily identify the brainstem subdivisions from which each section is taken. You should also be able to identify each of the cranial nerve nuclei (except for the four mentioned in the preceding paragraph). To consolidate your understanding of the surface anatomy of the brainstem and of sections through it, identify the point at which each cranial nerve enters or leaves the brain, and determine the approximate trajectory of the axons as they travel to or from this point to the cranial nerve nuclei with which they are connected. By the end of this laboratory experience, you should be able to view a cross section through the brainstem and identify the landmarks that characterize the subdivision, the level of the brainstem from which it was taken and the locations of the cranial nerves and nuclei that are present. Make sure that you can identify all of the structures (in bold) discussed in this chapter and identified in the figures.
Signs and symptoms associated with specific lesions of the brainstem

Figure 3.8 contains examples of four different lesions that are commonly seen after vascular accidents involving the brainstem. Consider each of these sections in turn, and work through Challenge 3.1: predicting the functional impairments that result from stroke in the perforating and circumferential vessels that supply the brainstem.
Challenge 4.1—predicting functional impairment post stroke

Selective occlusion or hemorrhage of specific arteries and arterioles that supply the brainstem can lead to damage that is preferentially localized to its medial or lateral parts. This is because, as detailed in Lab 1, the vascular supply to the medial brainstem is distinct from that to the lateral brainstem. Using the information in Labs 1 & 2, as well as the sections in Sylvius4 Online, identify which cranial nerve structures are likely to be damaged in each of the lesions indicated in Figure 3.8. Once you identify the nerves involved, discuss among your teammates what functional impairments are likely associated with each of these brainstem strokes. |
Neuromodulatory nuclei of the brainstem
In contrast to the cranial nerve nuclei that mediate particular unimodal sensory or motor functions, other nuclei of the brainstem have widespread projections throughout the central nervous system and serve to “modulate” neural activities, rather than “drive” neuronal output. Neurons in these modulatory nuclei synthesize and secrete a variety of small molecule neurotransmitters, including biogenic amines and acetylcholine, as well as a diverse set of neuropeptide transmitters. The actions of these neurotransmitters are typically mediated by G-protein coupled (metabotropic) receptors and are more slowly developing and long-lasting than the ionotropic actions of conventional fast neurotransmitter systems (e.g., glutamate and GABA). This is the principal reason why these widespread projection systems are considered “neuromodulatory” in nature.
Neuromodulatory systems are implicated in a wide range of behaviors (ranging from central homeostatic functions to cognitive phenomena, such as attention). It is therefore not surprising that dysregulation of the neuromodulatory systems—the biogenic amine neurotransmitters in particular—are implicated in most disorders of human behavior. It should also be of no surprise that many drugs of abuse act on these same biogenic amine pathways. The pharmacology of amine synapses is critically important in psychiatry, with drugs affecting the synthesis, receptor binding, or catabolism of these neurotransmitters being among the most important agents in the armamentarium of modern neuropharmacology.
Here, we will focus on four systems that can have profound effects on conscious states, cognition, and emotion: the mesencephalic dopamine systems, the pontine adrenergic and cholinergic systems, and the serotonergic systems of the pons and medulla. In the laboratory phase of this session, you will clearly see with the unaided eye evidence of the dopaminergic and adrenergic nuclei. You will see the general area where cholinergic and serotonergic nuclei are localized, but they will not be as clearly distinguishable.
Dopamine
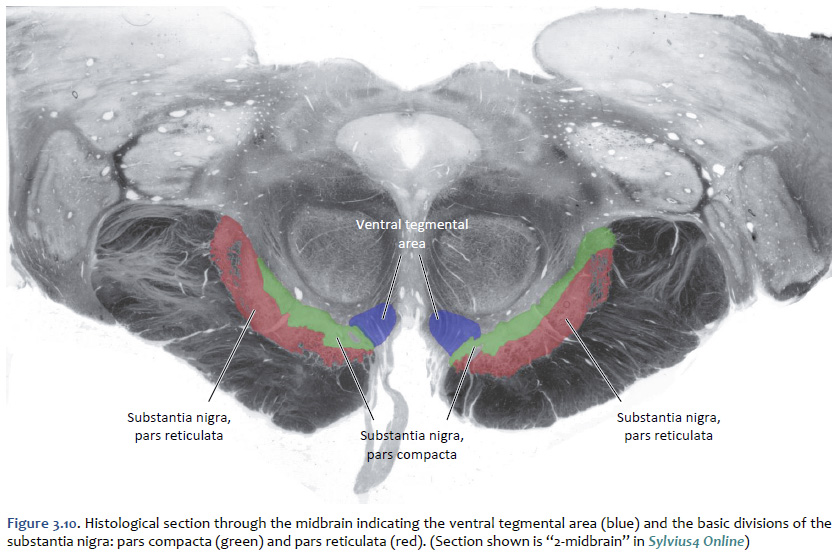
The cell bodies of the neurons that release dopamine as a neuromodulator are localized mainly to the ventral midbrain (mesencephalon), where they are organized into two principle divisions: a more loosely organized medial ventral tegmental area and a more tightly organized division of the substantia nigra known as the substantia nigra, pars compacta. The general distribution of projections from these divisions is illustrated in Figure 3.9. The locations of the cell bodies of origin of these projections is shown in Figure 3.10.
It is useful to differentiate three main systems of dopaminergic projections that emanate from these mesencephalic dopaminergic nuclei to the forebrain. Since they are all directed toward medial
- Mesostriatal or nigrostriatal: projections from the substantia nigra, pars compacta to the striatum, which is a principal input division of the basal ganglia (to be discussed in Lab 4)
- Mesolimbic: projections from the ventral tegmental area to components of the limbic forebrain (see Appendix 4), including the ventral division the striatum, known as the nucleus accumbens
- Mesocortical: projections mainly from the ventral tegmental area to the prefrontal cortex.
The functions of these three streams of dopaminergic projections to the forebrain will be discussed in the behavioral science sessions of our course on Brain and Behavior. For now, suffice it to summarize their functions as follows:
- Mesostriatal or nigrostriatal: facilitation of motor program selection and movement initiation. An important degenerative condition that we will study elsewhere is Parkinson’s disease, which results from the loss of mesencephalic dopaminergic neurons. The cardinal signs associated with Parkinson’s disease (i.e., bradykinesia) are attributable to loss of the nigrostriatal projection.
- Mesolimbic: facilitates the activation of reward circuitry in the limbic forebrain and prefrontal cortex. Plasticity in this projection has been implicated in addiction and the expression of addictive behavior. In a different context, over activity of the mesolimbic pathway is thought to be important in the “positive” symptoms associated with psychosis and schizophrenia, such as hallucinations.
- Mesocortical: facilitates executive functions in prefrontal lobe circuitry, including working memory, behavior choice, and attentional aspects of motor initiation. Dysfunction or injury to the mesocortical dopaminergic pathway may contribute to the cognitive deficits seen in Parkinson’s disease, and in the “negative” symptoms associated with schizophrenia.
The mesencephalic dopaminergic nuclei are visible to the unaided eye in gross specimens by the presence of a dark pigment named neuromelanin, which accumulates across the lifespan in the cell bodies of catecholaminergic neurons (Figure 3.11). Neuromalanin is a polymer made from 5,6-dihydroxyindole monomers; evidently, its accumulation is benign with respect to the metabolic and biosynthetic activities of catecholaminergic neurons. It is this pigment that gives the appearance of “dark substance” in the pars compacta division of the substantia nigra and in the ventral tegmental area (“substantia nigra” means “dark substance”). At autopsy, the brain from an individual with idiopathic Parkinson’s disease would be expected to exhibit far less “dark substance” due to the degeneration of the dopaminergic neurons.

In addition to the mesencephalic dopaminergic neurons, dopaminergic neurons with more specialized functions are localized to the retina, the olfactory bulbs, the hypothalamus (where they function to inhibit prolactin release from the anterior pituitary), and the medulla.
Norepinephrine
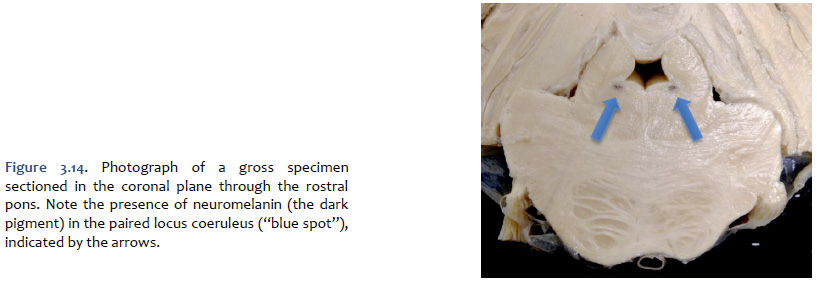
The cell bodies of the neurons that release norepinephrine (noradrenaline) as a neuromodulator in the brain are localized mainly to a distinctive, needle-shaped nucleus of the rostral pons called the locus coeruleus. However, there are also noradrenergic neurons scattered in the lateral tegmentum of the pons and medulla. Ascending noradrenergic projections from the locus coeruleus (and lateral tegmentum) reach the entire forebrain (Figure 3.12). The location of the locus coeruleus is indicated in Figure 3.13.
The widespread projections of the locus coeruleus (and lateral tegmentum) influences sleep and wakefulness, attention, and feeding behavior. They also contribute to transient stress-related responses helping to mediate a heightened state of vigilance and synergizing with sympathetic activation of visceral motor effectors. In the cerebral cortex, the effects of noradrenaline can be suppressive or facilitative (depending on the receptors expressed by postsynaptic structures), but in the thalamus, the effects generally are facilitative. Noradrenaline, together with serotonin, appears to have a role to play in the central modulation of pain in the brainstem and spinal cord. It also is implicated in mood disorders, such as depression and bipolar disorder, and in a variety of anxiety disorders.
Like dopaminergic neurons, the noradrenergic neurons of the locus coeruleus accumulate neuromelanin, which gives the locus coeruleus is characteristic “blue spot” appearance (“locus coeruleus” means “blue spot”). Although, in most brain specimens sectioned grossly through the pons, the pigment coloration of the locus coeruleus is indistinguishable to the unaided eye from the substantia nigra, pars compacta (Figure 3.14).
Serotonin (5-hydroxytryptamine, or "5-HT")

Serotonin is found primarily in groups of neurons in the median “seam” of the pons and rostral medulla; these neurons are organized into narrow midline nuclei called the raphe nuclei (“raphe” means “seam”). The raphe nuclei have widespread projections throughout the central nervous system (Figure 3.15). The location of the pontine raphe is indicated in Figure 3.13.
Serotonin occupies a place of prominence in neuropharmacology because a large number of antipsychotic drugs that are valuable in the treatment of depression and anxiety act on serotonergic pathways. Many antidepressant drugs are selective serotonin reuptake inhibitors (SSRIs) that inhibit the up-take of serotonin by specific presynaptic transporters. Serotonin has been shown to modulate a variety of behaviors, including emotion, circadian rhythms, motor behaviors, and states of arousal. Impairments in the function of serotonin receptors have been implicated in numerous psychiatric disorders, such as depression, anxiety disorders, and schizophrenia. Activation of serotonin receptors also mediates satiety and decreased food consumption, which is why serotonergic drugs may be useful in treating eating disorders.
Acetylcholine

Acetylcholine (ACh) was the first substance identified as a neurotransmitter. In addition to the function of ACh as the neurotransmitter at skeletal neuromuscular junctions, as well as at the neuromuscular synapse between the vagus nerve and cardiac muscle fibers, ACh serves as a transmitter at synapses in the ganglia of the visceral motor system and at a variety of sites within the central nervous system. Although its actions in the brain are not as well understood as they are in the periphery, ACh does seem to function as a neuromodulator at central cholinergic synapses generally serving to increase the depolarizing response of neurons to glutamatergic inputs.
Cholinergic neurons with widespread projections are found mainly in two locations in the brain: in the ponto-mesencephalic junction of the brainstem, and in the basal forebrain. Neurons of the brainstem cholinergic projection system are found mainly in the lateral dorsal tegmental nuclei (see Figure 3.13) and the nearby peduncolopontine tegmental nucleus. Neurons in these nuclei give rise to projections that diffusely innervate so-called non-specific nuclei of the thalamus, which in turn project to widespread regions of the cerebral cortex where they have a general effect promoting arousal. In addition to their role in arousal, these cholinergic tegmental nuclei also play a role in the function of motor systems, maintaining widespread connections to the basal ganglia, the superior colliculus, the deep nuclei of the cerebellum, and to premotor circuits distributed throughout the brainstem and spinal cord.
Direct cholinergic projections to the cerebral cortex arise from neurons in the nucleus basalis (of Meynert), which is a component of the basal forebrain (identified in Lab 4). Other cholinergic projections in the forebrain come from the medial septal nuclei and the nucleus of the diagonal band (of Broca)—also components of the basal forebrain; these are mainly directed toward the hippocampus in the medial temporal lobe.
In addition to cholinergic neurons with long-ranging projections, the central nervous system also contains cholinergic interneurons with more short-range local connections. Among the more important clinically are the large, cholinergic interneurons of the striatum, an important division of the basal ganglia that you will identify in Lab 4 and study elsewhere in the course.
|